Saprotrophic nutrition: Difference between revisions
m The LinkTitles extension automatically added links to existing pages (<a rel="nofollow" class="external free" href="https://github.com/bovender/LinkTitles">https://github.com/bovender/LinkTitles</a>). |
m The LinkTitles extension automatically added links to existing pages (https://github.com/bovender/LinkTitles). |
||
(6 intermediate revisions by the same user not shown) | |||
Line 1: | Line 1: | ||
[[File:Saprobes.jpg|150px|thumb|right|Some Shaggy Pholiota growing on trees, these fungi are wood-rotting saprobes [7].]]'''Saprotrophic nutrition''' is an example of [[extracellular digestion]] of decayed organic matter by [[organisms]] such as fungi and [[soil bacteria]]. Saprotrophic microscopic fungi are often called '''saprobes'''; saprotrophic plants or bacterial flora are called '''saprophytes'''. The process is most often facilitated by active transport of such materials through endocytosis within the internal mycelium and its constituent hyphae. Fungi, bread mould, some protists, and many bacteria are saprophytic in nutrition. Saprobes often use their hyphae to penetrate wood and other solid materials. Saprotrophic bacteria are more adept at breaking down fluid and semi-fluid materials [2]. | [[File:Saprobes.jpg|150px|thumb|right|Some Shaggy Pholiota growing on trees, these fungi are wood-rotting saprobes [7].]]'''Saprotrophic nutrition''' is an example of [[extracellular digestion]] of decayed [[Organic Matter|organic matter]] by [[organisms]] such as fungi and [[soil bacteria]]. Saprotrophic microscopic fungi are often called '''saprobes'''; saprotrophic plants or bacterial flora are called '''saprophytes'''. The process is most often facilitated by active transport of such materials through endocytosis within the internal mycelium and its constituent hyphae. Fungi, bread mould, some protists, and many bacteria are saprophytic in nutrition. Saprobes often use their hyphae to penetrate wood and other solid materials. Saprotrophic bacteria are more adept at breaking down fluid and semi-fluid materials [2]. | ||
==Process== | ==Process== | ||
In the presence of decaying organic matter, saprophytes and saprobes release digestive enzymes in their surrounding medium to convert complex organic molecules down to their simpler constituents. This simpler food is then absorbed through the body surface and utilized for various metabolic activities in the saprophyte. An example of this is the breakdown of starch into glucose or sucrose [1]. | In the presence of decaying [[Organic Matter|organic matter]], saprophytes and saprobes release digestive enzymes in their surrounding medium to convert complex organic molecules down to their simpler constituents. This simpler food is then absorbed through the body surface and utilized for various metabolic activities in the saprophyte. An example of this is the breakdown of starch into glucose or sucrose [1]. | ||
*Proteins are broken down into their amino acid components via the breaking of peptide bonds | *Proteins are broken down into their amino acid components via the breaking of peptide bonds | ||
Line 20: | Line 20: | ||
===Materials Colonized by Saprotrophic Organisms [2]=== | ===Materials Colonized by Saprotrophic Organisms [2]=== | ||
====Wood==== | ====Wood==== | ||
Fungi are the fundamental decomposers of wood. Without the [[ecosystem services]] provided by fungi as wood decomposers, our forests would be filled with wood piles. There are two physiological types of wood-decay fungi recognized by those who work in the field: those that produce brown rots and those that produce white rots. These two physiological categories reflect the composition of the wood and the processes by which the fungi decompose the wood. Glucose stored in the wood is a vital nutrient for fungi that is sometimes difficult to obtain due to its binding in cellulose and lignin. Those fungi that digest the cellulose within the lignin matrix leave behind a mass of brown lignin. Those fungi that cause white rot dissolve the lignin first and leave behind the white fibrous cellulose for later digestion. The most efficient wood-decay fungi are members of the [[Basidiomycota]] and can be seen on decaying logs, stumps, and trees. | Fungi are the fundamental [[decomposers]] of wood. Without the [[ecosystem services]] provided by fungi as wood decomposers, our forests would be filled with wood piles. There are two physiological types of wood-decay fungi recognized by those who work in the field: those that produce brown rots and those that produce white rots. These two physiological categories reflect the composition of the wood and the processes by which the fungi decompose the wood. Glucose stored in the wood is a vital nutrient for fungi that is sometimes difficult to obtain due to its binding in cellulose and [[lignin]]. Those fungi that digest the cellulose within the lignin matrix leave behind a mass of brown lignin. Those fungi that cause white rot dissolve the lignin first and leave behind the white fibrous cellulose for later digestion. The most efficient wood-decay fungi are members of the [[Basidiomycota]] and can be seen on decaying logs, stumps, and trees. | ||
[[File:leaflitterfungi.jpg|thumb|right|150px|Mycelium (thread-like fungal structures) on leaf litter [9].]] | [[File:leaflitterfungi.jpg|thumb|right|150px|Mycelium (thread-like fungal structures) on leaf litter [9].]] | ||
====Fallen Leaves/Leaf Litter==== | ====Fallen Leaves/Leaf Litter==== | ||
Line 26: | Line 26: | ||
[[File:wrackdecomposers.png|thumb|left|200px|(left) ''Dendryphiella salina'', (center) ''Sigmoidea marina'', (left) ''Acremonium fuci'' [10].]] | [[File:wrackdecomposers.png|thumb|left|200px|(left) ''Dendryphiella salina'', (center) ''Sigmoidea marina'', (left) ''Acremonium fuci'' [10].]] | ||
====Wrack==== | ====Wrack==== | ||
Wrack is biomass consisting of vegetable matter made up of a variety of species such as algae, seagrass and some land plants that may have washed up on beaches. It is very high in nutrients and has been used by humans as a fertilizer. While wrack is highly nutritious, its chemical components are rather unusual and not typically found outside of marine ecosystems. Because of this, the fungi associated with the [[decomposition]] of wrack are also found in marine habitats. ''Dendryphiella salina'' is the chief fungi that decomposes wrack and can be found worldwide. It is a slow-growing mould that is often found in the company of ''Sigmoidea marina'' and ''Acremonium fuci''. Species of the [[ascomycete]] family Halosphaeriaceae are able to wait for wrack on beaches by attaching themselves to [[sand]] grains [[File:dungfung.jpg|thumb|200px|left|''Cheilymenia fimicola'', a tiny disk-like dung loving fungi. Partial to cow pats distributed across England and Ireland [11].]] and releasing spores with bristle-like appendages that keep them suspended in seafoam. | Wrack is biomass consisting of vegetable matter made up of a variety of species such as [[algae]], seagrass and some land plants that may have washed up on beaches. It is very high in nutrients and has been used by humans as a fertilizer. While wrack is highly nutritious, its chemical components are rather unusual and not typically found outside of marine ecosystems. Because of this, the fungi associated with the [[decomposition]] of wrack are also found in marine habitats. ''Dendryphiella salina'' is the chief fungi that decomposes wrack and can be found worldwide. It is a slow-growing mould that is often found in the company of ''Sigmoidea marina'' and ''Acremonium fuci''. Species of the [[ascomycete]] family Halosphaeriaceae are able to wait for wrack on beaches by attaching themselves to [[sand]] grains [[File:dungfung.jpg|thumb|200px|left|''Cheilymenia fimicola'', a tiny disk-like dung loving fungi. Partial to cow pats distributed across England and Ireland [11].]] and releasing spores with bristle-like appendages that keep them suspended in seafoam. | ||
====Dung==== | ====Dung==== | ||
Like wrack, dung is highly nutritious and full of useful compounds for the growth of organisms. Unlike wrack, dung supports a vast array of fungi, each highly adpted to finding and colonizing it. There are several ways that this is accomplished, ways that may involve very complex steps. There exist a wide variety of [[coprophilous]] saprophytes and saprobes. These dung loving organisms are diverse in their distribution, physiology and dung preference. Coprophilous fungi disperse spores into the air that can stick to herbivore food sources, the spores are then digested by the herbivores which in turn begins their germination stage. Some fungi do not disperse their spores away from the dung and instead produce slimy spores that adhere to the bodies of [[foraging]] flies, beetles and [[mites]]. These foragers then become hosts to the spores and carry them to the next dung pile where the fungi begins anew. | Like wrack, dung is highly nutritious and full of useful compounds for the growth of organisms. Unlike wrack, dung supports a vast array of fungi, each highly adpted to finding and colonizing it. There are several ways that this is accomplished, ways that may involve very complex steps. There exist a wide variety of [[coprophilous]] saprophytes and saprobes. These dung loving organisms are diverse in their distribution, physiology and dung preference. Coprophilous fungi disperse spores into the air that can stick to herbivore food sources, the spores are then digested by the herbivores which in turn begins their germination stage. Some fungi do not disperse their spores away from the dung and instead produce slimy spores that adhere to the bodies of [[foraging]] flies, beetles and [[mites]]. These foragers then become hosts to the spores and carry them to the next dung pile where the fungi begins anew. | ||
Line 32: | Line 32: | ||
==Saprobes as Symbionts== | ==Saprobes as Symbionts== | ||
In boreal forest ecosystems, as in most terrestrial ecosystems, micro-organisms play a fundamental role in the cycling of carbon and nutrients. In the acid mor soils of the boreal region, fungi are believed to be the main decomposer organisms. Some saprobes have been found to provide essential nutrients to certain plants that do not produce their own sources of metabolic carbon. One example of this are the tropical [[mycotrophic]] orchids of Asia called ''Epipogium roseum'' [3]. In a 2009 study, it was found that the presence of saprotrophic fungi reduced the concentration of phosphorous and calcium in the needles of Norway spruce trees (''Picea abies''). Another result of the study was an observed reduction in the ratio of waxes to carotenoids in the needles [4]. Furthermore, the acquisition of relatively simple organic nitrogen sources by ''P. resinosa'' is likely to be largely indirect via [[Ectomycorrhizal Fungi|ectomycorrhizal fungi]]. More complex organic nitrogen sources may become accessible to [[Ectomycorrhizal Fungi|ectomycorrhizal fungi]] (and thus possibly their host plants) following mineralization by saprotrophic fungi such as ''Penicillium'' or ''Trichoderma'' when carbon to nitrogen ratios are sufficiently low [5]. Thus, if the saprotrophic microfungi were able to mineralize N from organic sources that are less directly accessible to [[Ectomycorrhizal Fungi|ectomycorrhizal fungi]], one might predict the existence of either additive or synergistic interactions between coexisting microfungi and ectomycorrhizal fungi in terms of host plant N or P content. | In boreal forest ecosystems, as in most terrestrial ecosystems, micro-organisms play a fundamental role in the cycling of carbon and nutrients. In the acid mor soils of the boreal region, fungi are believed to be the main decomposer organisms. Some saprobes have been found to provide essential nutrients to certain plants that do not produce their own sources of metabolic carbon. One example of this are the tropical [[mycotrophic]] orchids of Asia called ''Epipogium roseum'' [3]. In a 2009 study, it was found that the presence of saprotrophic fungi reduced the concentration of phosphorous and calcium in the needles of Norway spruce trees (''Picea abies''). Another result of the study was an observed reduction in the ratio of waxes to carotenoids in the needles [4]. Furthermore, the acquisition of relatively simple organic nitrogen sources by ''P. resinosa'' is likely to be largely indirect via [[Ectomycorrhizal Fungi|ectomycorrhizal fungi]]. More complex organic nitrogen sources may become accessible to [[Ectomycorrhizal Fungi|ectomycorrhizal fungi]] (and thus possibly their host plants) following mineralization by saprotrophic fungi such as ''Penicillium'' or ''Trichoderma'' when carbon to nitrogen ratios are sufficiently low [5]. Thus, if the saprotrophic microfungi were able to mineralize N from organic sources that are less directly accessible to [[Ectomycorrhizal Fungi|ectomycorrhizal fungi]], one might predict the existence of either additive or synergistic interactions between coexisting microfungi and [[Ectomycorrhizal Fungi|ectomycorrhizal fungi]] in terms of host plant N or P content. | ||
==References== | ==References== | ||
Line 45: | Line 45: | ||
5. Wu, T., Z. Kabir, R. T. Koide. 2005. A possible role for saprotrophic microfungi in the N nutrition of ectomycorrhizal Pinus resinosa. Soil Biology and Biochemistry Volume 37: 965-975. [https://www.sciencedirect.com/science/article/pii/S0038071704004110] | 5. Wu, T., Z. Kabir, R. T. Koide. 2005. A possible role for saprotrophic microfungi in the N nutrition of ectomycorrhizal Pinus resinosa. Soil Biology and Biochemistry Volume 37: 965-975. [https://www.sciencedirect.com/science/article/pii/S0038071704004110] | ||
6. Wu, T. 2011. Can ectomycorrhizal fungi circumvent the nitrogen mineralization for plant nutrition in temperate forest ecosystems?. Soil Biology and Biochemistry Volume 43: 1109-1117. [https://www.sciencedirect.com/science/article/pii/S0038071711000745] | 6. Wu, T. 2011. Can [[Ectomycorrhizal Fungi|ectomycorrhizal fungi]] circumvent the nitrogen mineralization for plant nutrition in temperate forest ecosystems?. Soil Biology and Biochemistry Volume 43: 1109-1117. [https://www.sciencedirect.com/science/article/pii/S0038071711000745] | ||
7. Shaggy pholiota fungi. Science Photo Library. [https://www.sciencephoto.com/media/14614/view] | 7. Shaggy pholiota fungi. Science Photo Library. [https://www.sciencephoto.com/media/14614/view] |
Latest revision as of 14:46, 1 May 2025
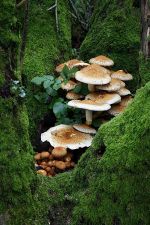
Saprotrophic nutrition is an example of extracellular digestion of decayed organic matter by organisms such as fungi and soil bacteria. Saprotrophic microscopic fungi are often called saprobes; saprotrophic plants or bacterial flora are called saprophytes. The process is most often facilitated by active transport of such materials through endocytosis within the internal mycelium and its constituent hyphae. Fungi, bread mould, some protists, and many bacteria are saprophytic in nutrition. Saprobes often use their hyphae to penetrate wood and other solid materials. Saprotrophic bacteria are more adept at breaking down fluid and semi-fluid materials [2].
Process
In the presence of decaying organic matter, saprophytes and saprobes release digestive enzymes in their surrounding medium to convert complex organic molecules down to their simpler constituents. This simpler food is then absorbed through the body surface and utilized for various metabolic activities in the saprophyte. An example of this is the breakdown of starch into glucose or sucrose [1].
- Proteins are broken down into their amino acid components via the breaking of peptide bonds
- Lipids are broken down into fatty acids and glycerol
- Starch is broken down into simpler sugars
Conditions
Optimal growth and repair of saprophytic organisms requires favorable conditions [1].
- Presence of water: 80–90% of the body of a fungi is comprised of water by mass. Fungi require excess water in their environment for intake due to the evaporation of internally retained water.
- Presence of oxygen: Anaerobic conditions do not allow for the optimal growth of saprophytic organisms above media such as soil and water.
- Neutral pH: pH levels around 7 are required.
- Moderate temperatures: Optimal growth of saprotrophic organisms occurs at 25°C, most of these organisms can grow and thrive between the temperatures of 1°C and 35°C.
Occurrence in Nature
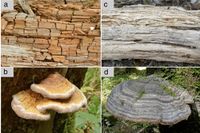
Materials Colonized by Saprotrophic Organisms [2]
Wood
Fungi are the fundamental decomposers of wood. Without the ecosystem services provided by fungi as wood decomposers, our forests would be filled with wood piles. There are two physiological types of wood-decay fungi recognized by those who work in the field: those that produce brown rots and those that produce white rots. These two physiological categories reflect the composition of the wood and the processes by which the fungi decompose the wood. Glucose stored in the wood is a vital nutrient for fungi that is sometimes difficult to obtain due to its binding in cellulose and lignin. Those fungi that digest the cellulose within the lignin matrix leave behind a mass of brown lignin. Those fungi that cause white rot dissolve the lignin first and leave behind the white fibrous cellulose for later digestion. The most efficient wood-decay fungi are members of the Basidiomycota and can be seen on decaying logs, stumps, and trees.
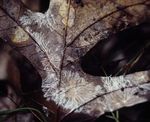
Fallen Leaves/Leaf Litter
Fallen leaves offer an abundant source of cellulose for forest ecosystems and their organisms. Some organisms begin their lives as endophytes and begin digesting the cellulose as soon as possible while other organisms occupy the leaves after they have fallen and begin rapidly digesting the cellulose. Leaves contain less lignin than wood so their soft parts are much easier to break down and digest. All types of leaves are colonized and decomposed by saprotrophic fungi.
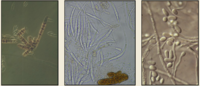
Wrack
Wrack is biomass consisting of vegetable matter made up of a variety of species such as algae, seagrass and some land plants that may have washed up on beaches. It is very high in nutrients and has been used by humans as a fertilizer. While wrack is highly nutritious, its chemical components are rather unusual and not typically found outside of marine ecosystems. Because of this, the fungi associated with the decomposition of wrack are also found in marine habitats. Dendryphiella salina is the chief fungi that decomposes wrack and can be found worldwide. It is a slow-growing mould that is often found in the company of Sigmoidea marina and Acremonium fuci. Species of the ascomycete family Halosphaeriaceae are able to wait for wrack on beaches by attaching themselves to sand grains
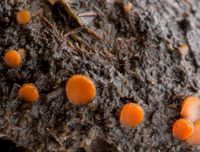
and releasing spores with bristle-like appendages that keep them suspended in seafoam.
Dung
Like wrack, dung is highly nutritious and full of useful compounds for the growth of organisms. Unlike wrack, dung supports a vast array of fungi, each highly adpted to finding and colonizing it. There are several ways that this is accomplished, ways that may involve very complex steps. There exist a wide variety of coprophilous saprophytes and saprobes. These dung loving organisms are diverse in their distribution, physiology and dung preference. Coprophilous fungi disperse spores into the air that can stick to herbivore food sources, the spores are then digested by the herbivores which in turn begins their germination stage. Some fungi do not disperse their spores away from the dung and instead produce slimy spores that adhere to the bodies of foraging flies, beetles and mites. These foragers then become hosts to the spores and carry them to the next dung pile where the fungi begins anew.
Saprobes as Symbionts
In boreal forest ecosystems, as in most terrestrial ecosystems, micro-organisms play a fundamental role in the cycling of carbon and nutrients. In the acid mor soils of the boreal region, fungi are believed to be the main decomposer organisms. Some saprobes have been found to provide essential nutrients to certain plants that do not produce their own sources of metabolic carbon. One example of this are the tropical mycotrophic orchids of Asia called Epipogium roseum [3]. In a 2009 study, it was found that the presence of saprotrophic fungi reduced the concentration of phosphorous and calcium in the needles of Norway spruce trees (Picea abies). Another result of the study was an observed reduction in the ratio of waxes to carotenoids in the needles [4]. Furthermore, the acquisition of relatively simple organic nitrogen sources by P. resinosa is likely to be largely indirect via ectomycorrhizal fungi. More complex organic nitrogen sources may become accessible to ectomycorrhizal fungi (and thus possibly their host plants) following mineralization by saprotrophic fungi such as Penicillium or Trichoderma when carbon to nitrogen ratios are sufficiently low [5]. Thus, if the saprotrophic microfungi were able to mineralize N from organic sources that are less directly accessible to ectomycorrhizal fungi, one might predict the existence of either additive or synergistic interactions between coexisting microfungi and ectomycorrhizal fungi in terms of host plant N or P content.
References
1. Clegg & Mackean (2006, p. 296), fig 14.17—A diagram explaining the optimal conditions needed for successful growth and repair
2. Malloch, D. 2017. Natural History of Fungi. A part of the Mycology Web Pages, New Brunswick Museum. <http://website.nbm-mnb.ca/mycologywebpages/NaturalHistoryOfFungi/Saprotrophs.html>. Downloaded on 28 April 2019. [1]
3. Selosse, M. A., F. Martos, B. Perry, P. Maj, M. Roy, T. Pailler. 2010. Saprotrophic fungal symbionts in tropical achlorophyllous orchids. Plant Signaling and Behavior Volume 5: 349-353. [2]
4. Mrnka, L., H. Tokárová, M. Vosátka, P. Matějka. 2009. Interaction of soil filamentous fungi affects needle composition and nutrition of Norway spruce seedlings. Trees Volume 23: 887-897. [3]
5. Wu, T., Z. Kabir, R. T. Koide. 2005. A possible role for saprotrophic microfungi in the N nutrition of ectomycorrhizal Pinus resinosa. Soil Biology and Biochemistry Volume 37: 965-975. [4]
6. Wu, T. 2011. Can ectomycorrhizal fungi circumvent the nitrogen mineralization for plant nutrition in temperate forest ecosystems?. Soil Biology and Biochemistry Volume 43: 1109-1117. [5]
7. Shaggy pholiota fungi. Science Photo Library. [6]
8. Fig. 1. Springer Link. [7]
9. Hyferne. [RED]. [8]
10. Wrack Fungi. THE SATROPHS. New Brunswick Museum. [9]
11. Dung Fungi. Alchetron. [10]