Soil hydrology
Soil hydrology is the study of the movement and distribution of water through soil and is a subset of the larger field of Hydrogeology. Hydrologic processes within soil include infiltration, storage, drainage, redistribution, evaporation, and transpiration. The structural properties of soil (composition, porosity, etc) and the chemical properties of water regulate the flow of water and other materials through soil.
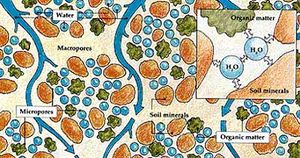
Physical Properties
Water Distribution in Soil
Liquid water is a key component of the three-phase (solid, liquid, gas) soil system, possibly occupying 50% or more of the total soil volume under saturated conditions. Even under relatively dry conditions, water can be held within soil pores, occupying approximately 5–10% of the soil volume. Particle size is strongly related to how much water soil can retain. Soils with smaller particle sizes, such as clay, will retain more water than soils with larger particles sizes, such as sand. Liquid water is held in soil due to the adhesive and cohesive forces associated with water's molecular structure.[2]
Three types of water exist in soils- gravitational, adsorbed, and capillary. Gravitational water easily moves through large pore spaces under the influence of gravity and tends not to to be accessible to plants because it moves quickly through the soil. Adsorbed water is held tightly against soil particles due to adhesion, which is the attractive force between water molecules and solid surfaces. This type of water also tends not to be accessible to plants because of the strong adhesive forces binding it to the surface of the soil particle. Capillary water is held in the spaces between soil particles and is generally the most accessible to plants. Capillary water occurs in soil pore spaces due to cohesion, or the attractive force between water molecules. The larger the pore spaces, the more water there is available for plants.
The interaction between water and soil particles is often visualized with a capillary tube model. Water molecules are more attracted to each other than to air (due to hydrogen bonding and cohesion), which creates surface tension. When combined with adhesion, or the attraction between water molecules and solid surfaces (the capillary tube wall), a meniscus is formed at the water-air interface. Water will rise in the capillary tube until it reaches an equilibrium between the attractive upward force at the interface and the weight of water pulling downward on the meniscus.[3] The capillary tube can be used to understand how water behaves in soil pores, and has also been used to understand how management practices such as agricultural tillage that alter pore size distribution can affect soil's ability to hold water.
Darcy's Law
The movement of liquids through porous media was first described mathematically by Henry Darcy in 1856 and is known as Darcy's law.[4]
Q = KA(dH/dL)
When applied to soils, Darcy's law states that the flow rate (Q) of water is equal to the hydraulic conductivity of the soil (K) times the cross-sectional area (A) times the hydraulic gradient (dH/dL), which is the change in head over the change in length of interest. This equation is commonly used when applying soil biogeochemical and hydraulic models.[5]
Temperature Regulation
Water within the soil system also influences terrestrial temperature dynamics. When water enters the soil matrix, it displaces gas in the soil. Water requires a relatively large energy input or loss (heat capacity, 4.18 kJ kg-1 K-1) to change its temperature because of strong interactions (hydrogen bonding) between water molecules. Alternately, weak inter-molecular interactions in soil gases allow temperatures to change readily with a small energy input or loss. In moist environments with ample soil water, water vapor loss from soil through evaporation requires a large energy input (heat of vaporization, 2449 kJ kg-1) without accompanying temperature change because of the large energy input required to disrupt the hydrogen bonds between liquid water molecules. Similarly, a large latent heat loss is required for soil freezing due to the high heat of fusion (334 kJ kg-1) associated with the more rigid, low-density structure of ice. Thermal properties of water within soil mediate environmental conditions for the biological community and regulate the thermodynamics of biogeochemical reactions.[6]
Chemical Properties
As a Solvent
The chemical properties of water govern its behavior in the environment and control many key processes occurring in soils as it interacts with organisms, minerals, and air spaces. As a result of its nonlinear structure and polarity, water is a powerful solvent, allowing it to readily dissolve ionic solids. Water molecules diminish the attractive force of ions by surrounding and forming solvation spheres around them. This allows water to stabilize the charges of both anions and cations, preventing their association.[7][8]
The ability of water to stabilize charged species in solution allows it to support the flow of electrons in soils. As such, water helps mediate oxidation and reduction (redox) reactions within the soil solution. Water itself may participate in these processes, and it is a product of cellular respiration in soils. In aerobic soils, water is produced from the oxidation of carbon in organic matter for energy production by microorganisms.
pH Regulation
Another particularly important chemical property of water is that it is amphoteric, meaning that it can act as either an acid or a base. Due to its polarity, water readily dissociates into protons and hydroxide ions. When it reacts with a strong base, water acts as an acid, releasing protons. When it reacts with a strong acid, water acts as a base, accepting protons. This amphoteric behavior of water facilitates the acid-base chemistry and dictates the potential pH range of aqueous solutions, thereby imparting soil pH — a "master variable" of soils that influences soil formation, plant growth, and environmental quality.
References
- ↑ http://www.fao.org/3/a0072e/a0072e07.htm
- ↑ Hillel, D., 1974. Soil and water. Physical principles and processes.
- ↑ Fisher, R.A., 1926. On the capillary forces in an ideal soil; correction of formulae given by W. B. Haines. J. Agric. Sci. 16, 492–505. https://doi.org/10.1017/S0021859600007838
- ↑ Darcy, H. (1856). Les fontaines publiques de la ville de Dijon. Paris: Dalmont.
- ↑ Hillel, D., 1998. Environmental soil physics. Academic Press, San Diego, CA.
- ↑ Campbell, G. S. & Norman, J. M. An Introduction to Environmental Biophysics. Vol. second 286 Springer-Verlag, 1998.
- ↑ Sparks, D. L. Environmental Soil Chemistry. 2nd ed. Academic Press, 2003.
- ↑ Eisenberg, D. & Kauzmann, W. The Structure and Properties of Water. 296 Oxford University Press, 1969.